Variable Displacement Linkage Pump/Motor (VDLP/M)

The MEPS group has designed a new architecture of variable displacement pump/motor, the variable displacement linkage pump/motor (VDLM/P). The VDLM/P uses an adjustable linkage with needle roller bearings to transmit force between a piston and a cam. This results in a machine with excellent volumetric and mechanical efficiency across a wide range of operating conditions, including low displacements (>90% total efficiency at 15% displacement). The VDLM/P can be designed as a low-speed high torque device, which uses a multi-lobe cam to achieve multiple piston strokes per revolution, or a high-speed motor with a single lobe cam or crankshaft. The VDLM/P is being consider for application from traction motors for off-highway vehicles to pressure washers to wave energy harvesting and has been designed with displacements from 8 cc/rev to 9,000 cc/rev.
Converting Wave Energy to Fresh Water

Wave energy is a widely available renewable energy source that can be harnessed by a wave energy converter, such as a buoy or oscillating flap. We are investigating using the power from a wave energy converter to drive a pump. The pressurized seawater can be used to drive a motor and an electric generator or as a feedwater source to make fresh, potable water using reverse osmosis. The MEPS lab is studying different architectures for the hydraulic power-take-off systems for these wave energy converters to make the systems as robust, cost effective, and efficient as possible.
Integrated Electric Motor - Hydraulic Pump

Hydraulic power transmission provides unmatched force and power density. The global push towards the electrification of machines with hydraulic systems creates the need for an integrated electric motor and hydraulic pump that is efficient and power dense. In collaboration with electric machine expertise in Eric Severson's group at University of Wisconsin - Madison , the MEPS group is developing a machine that integrates a radial piston hydrostatic pump with an axial flux electric motor. The integration of the electric motor and pump in a single casing eliminates redundant bearings, seals, and mechanical couplings, while allowing the pump leakage to directly cool the electric machine.
Soft Robots for Underground Burrowing
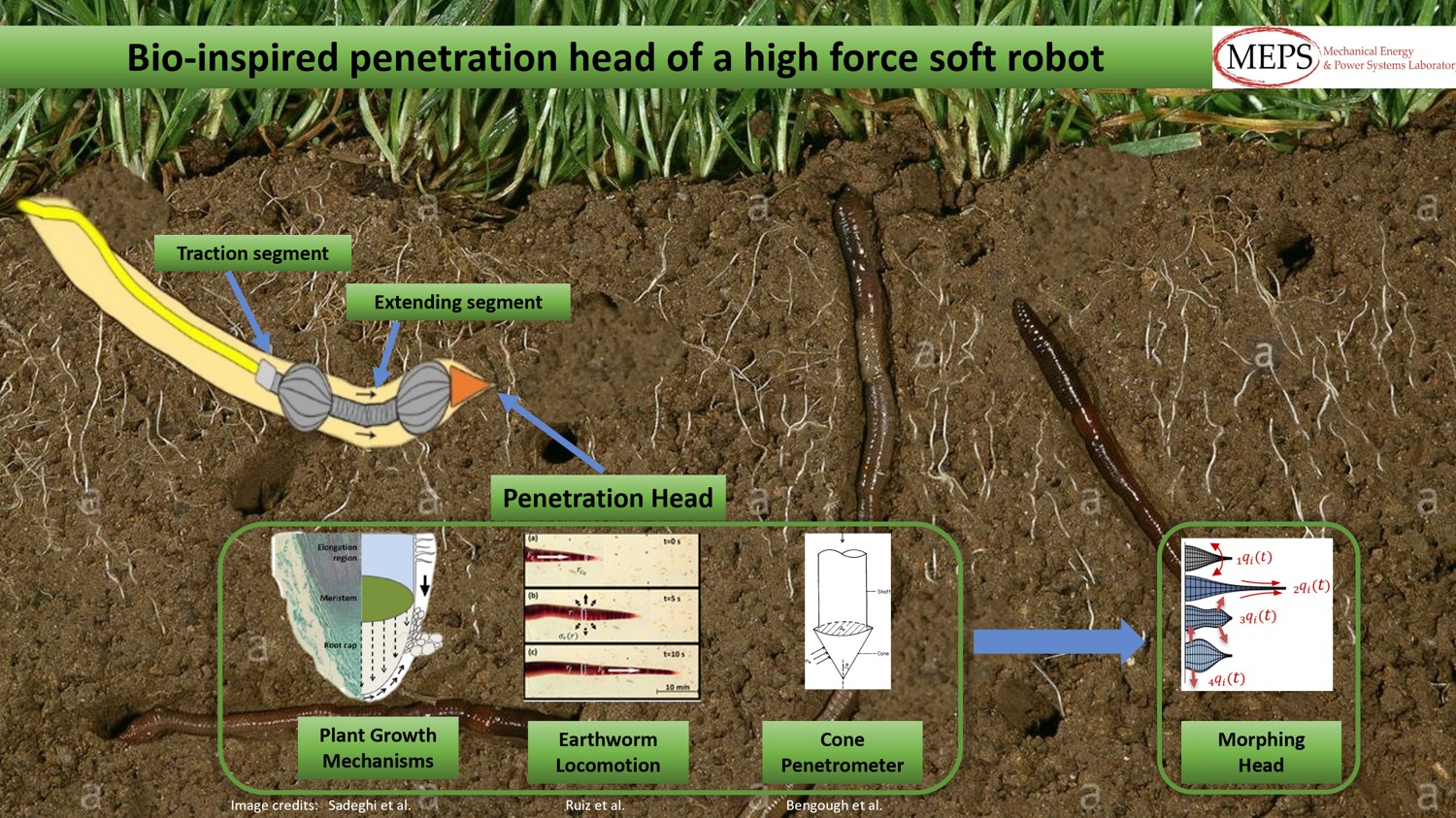
The MEPS group is designing a hydraulically powered soft robot for underground burrowing. The robot moves through the ground by alternately anchoring traction segments separated by an extending actuator. The robot forms the burrow with a morphing head that mimics the motion of an earthworm to plastically deform the soil and control the direction of travel.